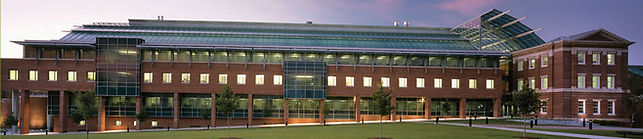


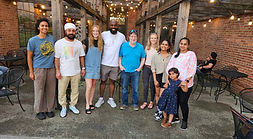

Enzyme-catalyzed Lactone Ring-Opening: A New Tool for Polymer Chemists
The role of enzymes in polymer synthesis and modification in novel environments has been a major research interest of the Gross Group. Enzyme-catalyzed lactone ring-opening polymerizations (ROP) offer important advantages relative to traditional synthetic methods. For example, enzyme-catalyzed lactone ROP simplifies stereochemical and regioselective control. The focus of our work has been to discover new enzyme-based pathways for polyester synthesis and to elucidate mechanisms involved in reactions. The systems studied have included ring-opening polymerizations using lipases to synthesize polyesters and polycarbonates. As a result of these studies, the range of lipase reactions has been significantly expanded and fundamental mechanisms involved in lipase-based ring-opening polymerizations for polyester and polycarbonate synthesis were illucidated. Selected examples of developments made in our laboratory on lipase-catalyzed ring-opening polymerizations are summarized below.
When we began this research program (1993), lipase-catalyzed polymerizations were slow and gave low molecular weights. For example, polymerizations using 20% lipase (w/w rel. to monomer) would require 4-days to reach 80% monomer conversions giving polymers with Mn about 2 000. Since then, our laboratory developed methods that transformed this work from an academic curiosity to a technology that now is under active development by our industrial partners. It is remarkable that lipase-catalyzed lactone ROPs can be conducted at temperatures from 20 to 108 oC, giving monomer conversions of >60 mol% within 2 h. An important step was identification of physically immobilized Lipase B from Candida Antarctica (immobilized CALB or Novozym 435, Novozymes Inc.).
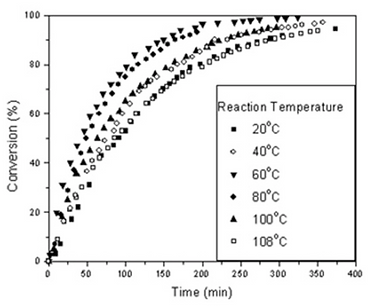
Figure 20: Monomer conversion as a function of time for ε-caprolactone polymerizations. The water content was kept constant (0.6 % by wt).
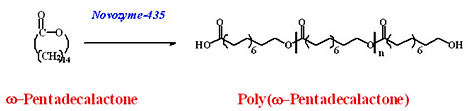
Scheme 15: CALB-catalyzed polymerization of ω-pentadecalactone (PDL) occurs rapidly (10-20 min) giving poly(PDL) in high molecular weight.
By using CALB (1% w/w relative to monomer), running reactions at high monomer concentrations, in low polarity medium, and limiting the number of initiation events by using low water concentration (e.g. 0.5% by wt), remarkable improvements were realized in monomer conversion efficiencies and product molecular weights (Kumar et al, 2000; Bisht et al, 1997). For example, e-caprolactone polymerizations at 70 oC that previously required 4-days to reach Mn 2 000 (see above) now only need 4 hours to give polycaprolactone (Mn 44 800 g/mol) in nearly quantitative yield. Polymerizations of macrolactones that proceed slowly by chemical methods were efficiently conducted by Novozyme-435 catalysis. Within 20 min, at 55 oC, polymer was formed in >90% yield with Mn 86 K (see Fig 21). The lack of sensitivity of enzyme-catalyzed lactone ROPs to oxygen, and tolerance to water (e.g. 0.6% w/w in reactions is typical), allows these reactions to be performed without the need for specialized reactors. Furthermore, we have shown unequivocally that for systems where monomer/polymer mixtures remain fluid to 90 oC, polymerizations can be conducted without addition of solvent. Poly(w-pentadecalactone) forms films with impressive properties. The films are best described as hard-tough with Tm 97 oC, Tg -27 oC, %-crystallinity ~60, and %-elongation ~650%. Further development of w-hydroxyfatty acids is underway with the aim to create efficient whole-cell biotransformations to these monomers. This will enable corresponding homo- and copolymers to be developed that have shown considerable promise as a diverse family of bio-based polyester materials.
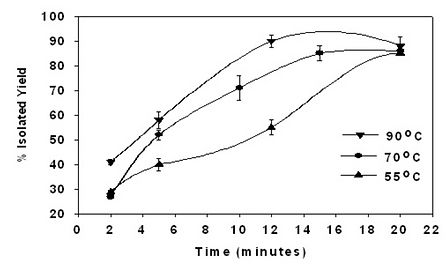
Figure 21. Novozym-435 catalyzed polymerization of ω-PDL (monomer to toluene 1:2 v/v). In only 12 minutes, poly(ω-PPDL) was formed with Mn up to 87 000 and Mw/Mn = 2.1.

Scheme 16: Revised mechanism by Mei et al (2003) of lipase catalyzed lactone ring-opening polymerization and competing reaction pathways.
Fundamental studies by our laboratory were performed to better understand the kinetics and mechanism(s) of lipase-catalyzed lactone ring-opening polymerizations. For porcine pancreatic lipase (PPL) catalyzed ε-caprolactone (ε-CL) polymerization in heptane, plots of log {[M]0/[M]t} vs time and Mn vs conversion to about 85% monomer conversion were constructed. The linearity of these two plots indicated termination and chain transfer did not occur. Therefore, the system provided “controlled” polymerizations where the molecular weight was a function of the monomer-to-initiator stoichiometry (Macdonald et al, 1995; Henderson et al, 1996)). The polymerization of ε-CL catalyzed by physically immobilized Lipase B from Candida antarctica (Novozyme-435) in bulk at 70 °C was also studied. We found that increased lipase concentration results in more rapid monomer conversion but decreased product molecular weight. A linear relationship between ln{([M]0−[M]i)/([M]t−[M]i)} vs reaction time and Mn vs monomer conversion (until 80%) was found. A key step in lipase-catalyzed polymerizations is formation of the acyl−enzyme intermediate.
Later, our laboratory (Mei et al, 2003) proposed a mechanism that expands on these findings by including formation of enzyme activated chain end complexes and intrachain scission reactions (Scheme 16).
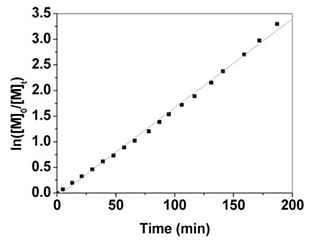
Figure 22. Semilogarithmic plot of Novozyme-435 catalyzed ε-caprolactone ring-opening polymerization at 60 °C, in toluene, with 1% (w/w) enzyme water content.

Figure 23. Dependence of molecular weight, Mn, upon monomer conversion of Novozyme-435 catalyzed ε-caprolactone ring-opening polymerization at 60 °C, in toluene, with 1% enzyme water content.
In addition, the revised mechanism described step-condensation reactions between activated chain ends and hydroxyl terminal groups. The inclusion of later steps in the mechanism was validated from inspection of Mn vs fractional ε-CL conversion plots at low enzyme water contents and high monomer conversion. An upward deviation from otherwise linear Mnvs fractional ε-CL conversion plots at the latter stage of the polymerization was seen (Fig 23). This was attributed to step-condensation reactions between enzyme-activated carboxyl chain ends and hydroxyl terminal groups.
Unlike previous studies, the kinetic plot of ln([M]0/[M]t) vs time was extended to 96% ε-CL conversion and Mn 11 970. By reaching this late stage in the polymerization, a sufficient effect was seen on Mn vs conversion plots to validate concurrent ring-opening and step-condensation reactions (Fig 22). By studying the effect of enzyme water content on the time course of ε-CL polymerization, plots of log{[M]0/[M]t} vs time, and ln kapp vs ln [enzyme], an experimental rate equation was derived that is zero order with respect to [R−OH] and initiator concentration, first order with respect to monomer concentration, and 0.7 order with respect to [enzyme], respectively. From an Arrhenius plot, an apparent activation energy of 2.88 kcal mol-1 was determined for Novozyme-435 catalyzed ε-CL polymerization in toluene. This value is well below 10.3 kcal mol-1 that is the activation energy for aluminum alkoxide catalyzed ε-CL polymerization in toluene.
References
-
Van As, B. A. C.; Thomassen, P.; Kalra, B.; Gross, R. A.; Meijer, E. W.; Palmans, A. R. A.; Heise, A. “One-Pot Chemoenzymatic Cascade Polymerization under Kinetic Resolution Conditions” Macromolecules; 37(24); 8973-8977 (2004).
-
Loeker, F. C.; Duxbury, C. J.; Kumar, R.; Gao, W.; Gross, R. A.; Howdle, S. M. Enzyme-Catalyzed Ring-Opening Polymerization of e-Caprolactone in Supercritical Carbon Dioxide. Macromolecules; 37(7); 2450-2453 (2004).
-
Mei, Y, Kumar, A, Gross; R.A., “Kinetics and Mechanism of Candida antarctica Lipase B Catalyzed Solution Polymerization of e-Caprolactone”, Macromolecules; 36(15); 5530-5536 (2003).
-
Bankova, M.; Kumar, A.; Impallomeni, G.; Ballistreri, A.; Gross, R. A.; “Mass-Selective Lipase-Catalyzed Poly(e-caprolactone) Transesterification Reactions”, Macromolecules; 35(18); 6858-6866, (2002).
-
Y. Mei, A. Kumar, and Richard A. Gross, “Probing Water-Temperature Relationships for Lipase-Catalyzed Lactone Ring-opening Polymerizations” Macromolecules; 35, 5444-5448 (2002).
-
R. Kumar and R.A. Gross, “Biocatalytic Route to Well-Defined Macromers Built around a Sugar Core, J. Am. Chem. Soc., 124(9): 1850-1851 (2002).
-
Kumar, R.A.Gross, J.D.Jenderoseck, “Poly(3-hydroxybutrate)-depolymerase from Pseudomonas Lemoignei: catalysis of esterification in organic media” Journal of Organic Chemistry, 65, 7800-7806 (2000).
-
A Kumar and R.A. Gross, “Candida antartica Lipase B Catalyzed Polycaprolactone Synthesis: Effects of Organic Media and Temperature, BioMacromolecules, 1, 133-138 (2000).
-
Kirpal S. Bisht, Lori A. Henderson, Richard A. Gross, David L. Kaplan and Graham Swift, “Enzyme-Catalyzed Ring-Opening Polymerization of Poly(ω-pentadecalactone)” Macromolecules, Vol. 30, 2705-2711 (1997).
-
Jin Xu, Stephen P. McCarthy and Richard A. Gross, “Racemic β-Methyl-α-propiolactone Polymerization by Organometallic Catalyst Systems”, Macromolecules, Vol. 29, No. 13, 4565-4571 (1996).
-
Jin Xu, Richard A. Gross, David L. Kaplan and Graham Swift, “Chemoenzymatic Synthesis and Study of Poly(β-methyl-α-propiolactone) Stereocopolymers”, Macromolecules, Vol. 29, No. 13, 4582-4590 (1996).
-
Jin Xu, Richard A. Gross, David L. Kaplan and Graham Swift, “Chemoenzymatic Route to Poly(3-hydroxybutyrate)”, Macromolecules, Vol. 29, 3857-3861 (1996).
-
Yuri Y. Svirkin, Jin Xu, Richard A. Gross, David L. Kaplan and Graham Swift, “Enzyme-Catalyzed Stereoselective Ring-Opening Polymerization of β-Methyl-α-propiolactone”, Macromolecules, Vol. 29, No. 13, 4591-4597 (1996).
-
Lori A. Henderson, Yuri, Y. Svirkin, Richard A. Gross, David L. Kaplan and -caprolactone: Effects ofεGraham Swift, “Enzyme Catalyzed Polymerizations of Initiator on Product Structure, Propagation Kinetics, and Mechanism”, Macromolecules, Vol. 29, 7759-7766 (1996).
-
Renée T. Macdonald, Satish K. Pulapura, Yuri Y. Svirkin and Richard A. Gross, "Enzyme Catalyzed ε-Caprolactone Ring-Opening Polymerization" Macromolecules, 28, 73-78 (1995).