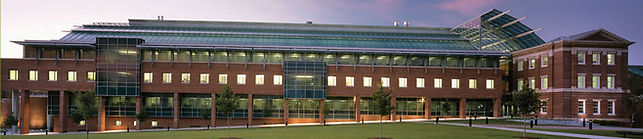


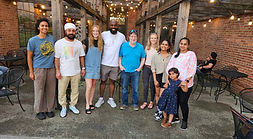

Influences of surface interactions and macro-porous matrix physical characteristics on the activity and thermal stability of Candida antarctica Lipase B
Our laboratory is exploring fundamental characteristics of Candida antarctica Lipase B (CALB)-surface interactions. While fundamental knowledge is lacking at a molecular level on how enzyme characteristics are influenced by contacts with surfaces, it is well known that shifts in enzyme activity and stability due to surface interactions can be dramatic. Adsorption of an enzyme onto a surface can induce conformational changes which affect the rate and specificity of the catalyst.
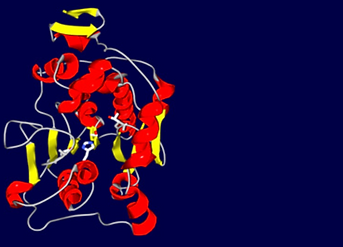
Scheme 14. Candida antarctica lipase B (CALB) Active site triad: Ser 105; Asp 187; His 224
To obtain a better understanding of how chemical and physical features of immobilization supports influence CALB properties, systematic changes were made in CALB loading, CALB distribution within immobilization supports, and the microenvironment that surrounds CALB. Previous studies generally did not consider enzyme distribution within carriers. However, this information is critical when seeking to better understand differences in enzyme activity observed by changing immobilization matrix parameters. For example, knowledge that an enzyme is primarily located within the outer regions of an immobilization support instead of being uniformly distributed throughout the matrix enables meaningful calculations of enzyme density along matrix surfaces as well as improved predictive models of substrate/product diffusion to and from immobilized protein.
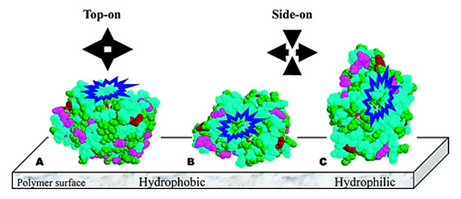
Figure 17. Three possible orientations of CALB: A, hydrophobic surface of CALB attached on hydrophobic films in top-on orientation; B, hydrophobic surface of CALB attached on films in side-on orientation; and C, hydrophilic surface of CALB attached on films in side-on orientation. The hydrophobic and hydrophilic amino acids are in blue and green, respectively. Cysteines are in pink. Other amino acids that can react with epoxy groups are in magenta. The blue loops represent entrances of active sites.
To determine CALB spatial distribution within support matrices, a synchrotron beam light source was used that provided spatial resolution to 5 by 5 mm. IR absorbance bands corresponding to CALB and the polymer matrix distinguished these two components and were used to generate semiquantitative maps of protein distribution throughout the matrix (Fig. 18). This method was first applied to define the protein distribution of Novozym 435, an important commercial immobilized CALB catalyzed produced by Novozymes (Ying et al., 2003).

Figure 19. Distribution of CALB as a function of the matrix diameter determined by IR microscopy. For images 1 and 2, bead diameter was 150 and 300 mm, respectively. Synchrotron light source was only used on the images of 3 (75 μm), 4 (35 μm), and 5 (35 μm).
Our studies found wide differences in CALB distribution within immobilization supports as a function of support matrix structure, pore and particle size. Generally, a more uniform distribution of CALB throughout matrices favored higher CALB activity (Nakaoki et al, 2005; Chen et al., 2007a; Chen et al., 2007b). Furthermore, acrylic polymers were synthesized where the ratio of hydrophobic to hydrophilic repeat units was systematically varied. These polymers containing glycidyl methacrylate units were spin coated to create flat surfaces for covalent immobilization of CALB and subsequent imaging by AFM (Chen et al, 2008). Increase in surface hydrophilic character resulted in CALB preferring to aggregate on surfaces, changes in enzyme conformation based on height measurements and decreased CALB specific activity (see Fig 17). The lessons learned provide a basis rationally design optimal immobilized enzyme CALB catalysts for low molar mass and polymerization reactions.
References
-
Chen, B.; Pernodet, N.; Rafailovich, M.H.; Bakhtina, A.; Gross, R.A. Protein Immobilization on Epoxy-Activated Thin Polymer Films: Effect of Surface Wettability and Enzyme Loading Langmuir, 2008, 24 (23), 13457-13464.
-
Bo Chen, Jun Hu, Elizabeth M. Miller, Wenchun Xie, Minmin Cai, and Richard A. Gross, Candida antarctica Lipase B Chemically Immobilized on Epoxy-Activated Micro- and Nanobeads: Catalysts for Polyester Synthesis Biomacromolecules , 9, 463-471(2008).
-
Bo Chen, Elizabeth M. Miller, Lisa Miller, John J. Maikner, and Richard A. Gross, Effects of Macroporous Resin Size on Candida antarctica Lipase B Adsorption, Fraction of Active Molecules, and Catalytic Activity for Polyester Synthesis Langmuir , 23, 1381-1387(2007a).
-
Chen, B.; Miller, M. E.; Gross, R. A., Effects of porous polystyrene resin parameters on Candida antarctica Lipase B adsorption, distribution, and polyester synthesis activity. Langmuir , 23 (11), 6467-6474 (2007b).
-
Mijovic, J.; Bian, Y.; Gross, R. A.; Chen, B. Dynamics of Proteins in Hydrated State and in Solution As Studied by Dielectric Relaxation Spectroscopy Macromolecules; 38(26) 10812-10819 (2005).
-
Nakaoki, T.; Mei, Y.; Miller, L.-M.; Kumar, A; Kalra, B; Miller, E.-M.; Kirk, O.; Christensen, M.; Gross, R.A. “Candida antartica Lipase B catalyzed polymerization of lactones: Effects of immobilization matrices on polymerization kinetics and Molecular Weight” Industrial Biotechnology; 1(2) 126-134 (2005).
-
Loos, K.; Kennedy, S. B.; Eidelman, N.; Tai, Y.; Zharnikov, M.; Amis, E. J.; Ulman, A.; Gross, R. A. Combinatorial Approach To Study Enzyme/Surface Interactions Langmuir; 21(12); 5237-5241 (2005).
-
Mei, Y.; Miller, L.; Gao, W.; Gross, R. A.; Imaging the Distribution and Secondary Structure of Immobilized Enzymes Using Infrared Microspectroscopy BioMacromolecules; 4(1); 70-74 (2003).
-
Dyal, A., Loos, Katja., Noto, M., Chang, S.W., Spagnoli, C., Shafi, Kurikka V.P.M., Ulman, A., Cowman,M.,Gross. R.A. Activity of Candida rugosa Lipase Immobilized on g-Fe2O3 Magnetic Nanoparticles J. Am. Chem. Soc. 125; 1684-1685 (2003).