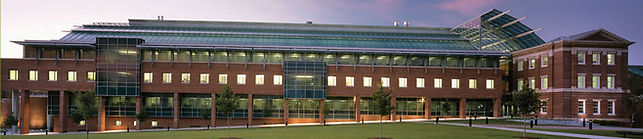


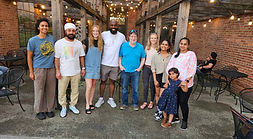

Sophorolipids, a microbial glycolipid
Our laboratory developed fermentative methods for the efficient synthesis of sophorolipids (SLs), a novel class of glycolipids. In one study, a simple and scalable fed-batch shake-flask method for the production of SLs by Candida bombicola ATCC 22214 is reported. Optimal aeration, expressed in terms of oxygen transfer rate, was between 50 and 80 mMO2/L h-1 and resulted in maximum values for both volumetric product formation (1-1.5 g/L h-1) and SL yield (350 g/L) (Guilmanov et al., 2002). Furthermore, in collaboration with Prof. Somasundaran at Columbia University, we began to explore selected colloidal properties of modified sophorolipids (Zhang et al., 2004). Modifications of SLs were performed so that the chain length of the n-alkyl group (methyl, ethyl, propyl, butyl, hexyl) esterified to the sophorolipid fatty acid was varied. The effect of the n-alkyl ester chain length on interfacial properties of corresponding sophorolipid analogues was studied.

Scheme 9: Structure of lactonic and acidic forms of sophorolipid mixture produced by Candida bombicola.
The cmc and minimum surface tension have an inverse relationship with the alkyl ester chain length. That is, cmc decreased to 1/2 per additional CH2 group for the methyl, ethyl, and propyl series of chain lengths. These results were confirmed by fluorescence spectroscopy. Adsorption of sophorolipid alkyl esters on hydrophilic solids was also studied to explore the type of lateral associations. These surfactants were found to absorb on alumina but much less on silica. This adsorption behavior on hydrophilic solids is similar to that of sugar-based nonionic surfactants and unlike that of nonionic ethoxylated surfactants. Hydrogen bonding is proposed to be the primary driving force for adsorption of the sophorolipids on alumina. Increase in the n-alkyl ester chain length of sophorolipids caused a shift of the adsorption isotherms to lower concentrations. The magnitude of the shift corresponds to the change in cmc of these surfactants. This study suggests that by careful modulation of the hydrophilic/lipophilic balance of sophorolipids via simple chemical modification, dramatic shifts in their surface-activity are achieved to ‘tune’ their properties for a desired interfacial challenge.
To fully explore the potential of sophorolipids to function in a variety of important areas requiring modulation of their physical and biological properties, our laboratory developed a series of chemo-enzymatic methods to vary sophorolipid structure. A mixture of sophorolipids produced by Torulopsis bombicola was esterified by reaction with sodium alcoxide. Using Novozym 435 as catalyst, SL alkyl esters in dry THF were reacted with vinyl acrylate and vinyl acetate to prepare diacyl derivatives. The reactions were highly regioselective, and exclusive acylation of hydroxyl groups on C-6ʹ and C-6ʺ took place (Bisht et al, 1999). The SL methyl ester, in the absence of the acylating agent, or with the agent at a concentration less than equimolar, gave sophorolactone.
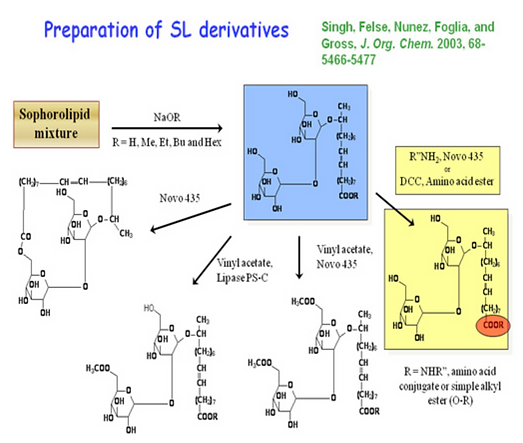
Scheme 10: Summary of chemo-enzymatic chemistry developed to prepare a library of sophorolipid analogs (see Azim et al., 2006; Singh et al., 2003; Bisht et al., 2000; Bisht et al. 1999).

Interestingly, spectral analysis of this compound showed that a synthetic analogue of microbially produced macrolactone was formed. Sophorolactone differs from the natural sophorolipid lactone in that the fatty acid carboxyl carbon is linked to the C-6ʺ hydroxyl, not to the C-4ʺ hydroxyl.
Subsequent acrylation of the new non-natural lactonic form of SL, catalyzed by Novozym 435, led to formation of the C-6ʹ monoacryl derivative (Bisht et al, 2000). Thus, a mono-acryl compound with potential to be used for introduction into polymers via free radical polymerization was synthesized. This series of sophorolipid analogues was further extended in a study by Singh et al (2003) to prepare a series of sophorolipid amides.
Figure 5. Metathesis ring-opening polymerization of natural lactonic sophorolipids (SL) gives poly(SL) with alternating C18 oleic acid and bulky
The translation of complexity from natural sophorolipid building blocks to polymers was explored. One approach was to directly polymerize lactonic sophorolipids derived from fermentation of Candida bombicola by ROMP catalysis. Poly(sophorolipid) was prepared in yield and Mn up to 89% and 103 000, respectively. By this chemo-biocatalytic route, unique polymers with disaccharide, ester, and monounsaturated hydrocarbon moieties were prepared.
In collaboration with Prof. Scandola at the University of Bologna studies were performed to better understand the solid state properties of poly(sophorolipids), poly(SL). The unique poly(SL) structure consists of C18 oleic-like aliphatic segments (90% cis-configured double bonds) that alternate with bulky diacetylated disaccharide moieties (Zini et al., 2008). Solid-state properties were investigated using TGA, DSC, TMDSC, and variable-temperature X-ray diffraction. Poly(SL) is a solid at room temperature that undergoes a glass transition at 61 °C and melts at 123 °C. The crystal phase is associated with ordered packing of aliphatic chain segments. Semicrystalline poly(SL) also displays a long-range order (d = 2.44 nm) involving sophorose groups that is found to persist after crystal phase melting (in high-T diffractograms) with a slightly shortened distance (2.27 nm). Upon annealing at 80 °C, poly(SL) recrystallizes and, concomitantly, the disaccharide units space out again at 2.44 nm. An exothermal phenomenon that immediately follows melting and is revealed by TMDSC might be associated with the observed adjustment of sophorose units spacing in the melt (Fig 6).
In collaboration with Prof. Zhou at CUNY Staten Island, our group has begun to explore self-assembly characteristics of natural sophorolipids (Zhou et al, 2004). Thus far, our work has focused on acidic sophorolipid (SL) molecules. They are bolaamphiphiles with unique structures that include an asymmetrical polar head size (disaccharide vs COOH), a kinked hydrophobic core (cis-9-octadecenoic chain), and a non-amide polar-nonpolar linkage. Light microscopy, small- and wide-angle X-ray scattering, FT-IR spectroscopy, and dynamic laser light scattering were used to investigate supramolecular structures of SL self-assembled aggregates at different pH values. In acidic conditions (pH<5.5), giant twisted and helical ribbons of 5-11 mm width and several hundreds of micrometers length were observed for the first time (Fig. 7). By increasing the solution pH, ribbon formation slowed, decreased in yield, ncreased in helicity and entanglements of the giant ribbons. An interdigitated lamellar packing model of acidic SL-COOH molecules with a long period of 2.78 nm, stabilized by both the strong hydrophobic association between cis-9-octadecenoic chains and strong disaccharide-disaccharide hydrogen bonding was proposed.
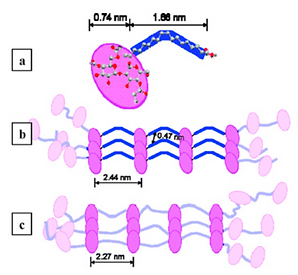
Figure 6. Poly(sophorolipid) packing model: molecular comformation of repeating unit (a) and sketch of a crystalline domain (b). Chain arrangement above the melting temperature (c).

Figure 7. Free acidic SL dissolved in dilute HCl, final pH = 4.1, C = 2.2 mg/mL. Big helical ribbons (e.g., ~11 mm wide and hundreds mm long) were observed
With the above established and a library of sophorolipid derivatives in hand, our laboratory, in collaboration with immunologists, virologists, and other medical experts, began studies to determine the potential of sophorolipid-based therapeutics. Experimental studies in mice and rats were performed to assess effects of sophorolipids on sepsis-related mortality when administered as a (1) single bolus versus sequential dosing and (2) natural mixture versus individual derivatives compared with vehicle alone (Bluth et al., 2006; Hardin et al., 2007). Intra-abdominal sepsis was induced in male, Sprague Dawley rats, 200 to 240 g, via cecal ligation and puncture. Sophorolipids (5–750 mg/kg) or vehicle (ethanol/sucrose/physiological saline) were injected intravenously (i.v.) via tail vein or inferior vena cava at the end of the operation either as a single dose or sequentially (q24 x 3 doses). The natural mixture was compared with select sophorolipid derivatives (n=10–15 per group). Sham-operated animals served as non-sepsis controls. Survival rates were compared at 1 through 6 d post sepsis induction.
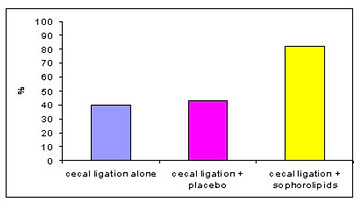
Figure 9. SLs improved the survival rate of Sprague Dawley rats who were in septic shock due to cecal ligation and puncture
The results showed sophorolipid treatment at 5 mg/kg body weight improved survival in rats with cecal ligation and puncture-induced septic shock by 28% at 24 h and 42% at 72 h for single dose, 39% at 24 h and 26% at 72 h for sequential doses, and 23% overall survival for select sophorolipid derivatives when compared with vehicle control (P < 0.05 for sequential dosing) (see Fig. 9). Toxicity was evident and dose-dependent with very high doses of sophorolipid (375–750 mg/kg body weight) with histopathology demonstrating interstitial and intra-alveolar edema with areas of micro-hemorrhage in pulmonary tissue when compared with vehicle controls (P < 0.05). No mortality was observed in sham operated controls at all doses tested. Therefore, it was concluded that administration of sophorolipids after induction of intra-abdominal sepsis improves survival. The demonstration that sophorolipids can reduce sepsis-related mortality with different dosing regimens and derivatives provides continuing evidence toward a promising new therapy.
Other work has shown that various members of our modified sophorolipid library have excellent potential for use as antibacterial, antiviral, and anti-inflammatory properties (Mueller et al, 2006; Shah et al., 2005). In one example, work with Bluth and colleagues at SUNY Downstate Medical Center has shown that sophorolipids are able to down-regulate expression of proinflammatory cytokines including interleukin (Hagler et al. 2007). Furthermore, Table 1 below shows how antibacterial properties of sophorolipids can be increased by up to 1000 times relative to the natural SL mixture by simple modifications of sophorolipids such as esterification of fatty acid carboxyl groups and selective acetylation of disaccharide hydroxyl groups.
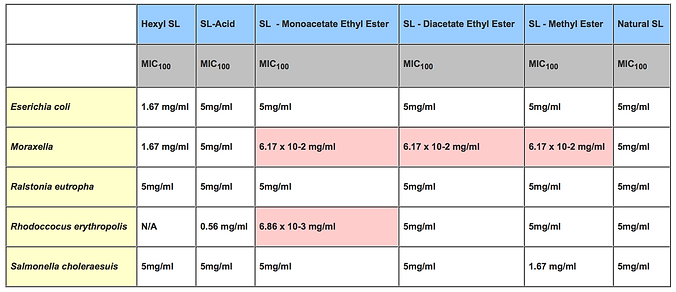
Table 1. Gram Negative Bacteria: MIC100 : Concentration of Sophorolipid Needed to Completely Inhibit Growth. N/A :No Inhibitory Effect Observed.
Studies were also performed in collaboration with the Eastern Virginia Medical School (Gustavo F. Doncel M.D.) to explore the dual use of sophorolipids for protection against the human immunodeficiency virus (HIV)/AIDS disease as well as their efficacy and safety as a vaginal topical microbicide (Shah et al, 2005). The sophorolipid diacetate ethyl ester derivative is the most potent spermicidal and virucidal agent of the series of SLs studied. Its virucidal activity against HIV and sperm-immobilizing activity against human semen are similar to those of nonoxynol-9. However, it also induces sufficient vaginal cell toxicity to raise concerns about its applicability for long-term microbicidal contraception. Further work is planned to further elucidate structure-activity relationship to create new sophorolipid analogs with less cytotoxicity and higher activity.

Figure 10. Cytotoxicity of sophorolipid and derivatives: HPAC cells were cultured with 100 g of sophorolipid natural mixture or select derivatives. Cytotoxicity was determined as described in Materials and methods. Data are presented as mean of three experiments and are reported as percent cytotoxicity ± SE and significance determined by Student’s t-test; *P≤ 0.05 compared with natural mixture (SL mix). SL mix = natural mixture; SL EE = ethyl ester; SL EEM = ethyl ester monoacetate; SL EED = ethyl ester diacetate; SL ME = methyl ester; AS = acidic sophorolipid; LSD = lactonic sophorolipid diacetate.
In collaboration with Martin Bluth M.D. (SUNY Downstate Medical school), our laboratories reported for the first time that sophorolipids mediate cytotoxic responses to pancreatic cancer cell lines (Fu et al., 2008). These anticancer responses were dose- and derivative-dependent and likely kill cancer cells by necrosis (see Fig 10 that illustrates SL structure-activity relationships with HPAC cells). Furthermore, these agents are specific to cancer cells in that they did not affect normal human cells. Hence, sophorolipids represent a unique and novel class of drugs, which have potential to be further tuned to increase their potency and specificity against pancreatic cancer. Further studies are needed to define the precise mechanisms involved (necrosis versus apoptosis) and if these mechanisms are derivative specific.
References
-
Fu SL, Wallner SR, Bowne WB, Hagler, MD; Zenilman, ME; Gross, R.A., Bluth, MH. Sophorolipids and their derivatives are lethal against human pancreatic cancer cells, Journal of Surgical Research 148 (1), 77-82 (2008).
-
Zini E, Gazzano M, Scandola M, Wallner, S.R. Gross, R. A. Glycolipid Biomaterials: Solid-State Properties of a Poly(sophorolipid). Macromolecules 42(20) 7463-7468 (2008).
-
Hardin, R.; Pierre, J.; Schulze, R.; Mueller, C. M.; Fu, S. L.; Wallner, S. R.; Stanek, A.; Shah, V.; Gross, R. A.; Weedon, J.; Nowakowski, M.; Zenilman, M. E.; Bluth, M. H., Sophorolipids improve sepsis survival: Effects of dosing and derivatives. Journal of Surgical Research, 142 (2), 314-319 (2007).
-
Fu, S. L.; Mueller, C.; Lin, Y. Y.; Viterbo, D.; Pierre, J.; Shah, V.; Gross, R.; Schulze, R.; Zenilman, M., Sophorolipid treatment decreases LIPS induced inflammatory responses and NO production in macrophages. Journal of the American College of Surgeons, 205 (3), S44-S44 (2007).
-
Hagler, M.; Smith-Norowitz, T. A.; Chice, S.; Wallner, S. R.; Viterbo, D.; Mueller, C. M.; Gross, R.; Nowakowski, M.; Schulze, R.; Zenilman, M. E.; Bluth, M. H., Sophorolipids decrease IgE production in U266 cells by downregulation of BSAP (Pax5), TLR-2, STAT3 and IL-6. Journal of Allergy and Clinical Immunology, 119 (1), S263-S263 (2007).
-
Felse, P. A.; Shah, V.; Chan, J.; Rao, K. J.; Gross, R. A., Sophorolipid biosynthesis by Candida bombicola from industrial fatty acid residues. Enzyme and Microbial Technology, 40 (2), 316-323 (2007).
-
Wei Gao, Rena Hagver, Vishal Shah, Wenchun Xie, Richard A. Gross, M. Firat Ilker, Chrissy Bell, Kelly A. Burke, and E. Bryan Coughlin Glycolipid Polymer Synthesized from Natural Lactonic Sophorolipids by Ring-Opening Metathesis Polymerization Macromolecules , 40(2), 145(2006).
-
Bluth, M.H.; Kandil, E; Mueller, C.M.; Shah, V.; Lin, Y.Y.; Zhang, H; Dresner, L.; Lempert, L.; Nowakowski, M.; Gross, R; Schulze, R.; Zenilman, M.E. Sophorolipids block lethal effects of septic shock in rats in a cecal ligation and puncture model of experimental sepsis, Critical Care Medicine 34 (1): 188-195 (2006).
-
Mueller, C.M.; Viterbo, D.; Murray, P.J.; Shah, V.; Gross, R.; Schulze, R.; Zenilman, M.E.; Bluth, M.H. Sophorolipid treatment decreases inflammatory cytokine expression in an in vitro model of experimental sepsis. Faseb Journal 20 (4): A204-A204 Part 1, (2006).
-
Azim, A; Shah, V.; Doncel, G.F.; Peterson, N.; Gao, W.; Gross, R. Amino acid conjugated sophorolipids: A new family of biologically active functionalized glycolipids. Bioconjugate Chemistry 17 (6): 1523-1529 (2006).
-
Shah V, Doncel GF, Seyoum T, Eaton KM, Zalenskaya I, Hagver R, Azim A, Gross R. Sophorolipids, microbial glycolipids with anti-human immunodeficiency virus and sperm-immobilizing activities. Antimicrob Agents Chemother; 149; 1-8 (2005).
-
Zhang, L., Somasundaran, P., Singh, S. K., Felse, A. P., Gross, R.A. Synthesis and interfacial properties of sophorolipid derivatives Colloids and Surfaces A:Physicochem.Eng. Aspects; 240; 75-82 (2004).
-
Zhou, S., Xu, Chang.., Wang, J., Gao, W., Akhverdiyeva., Shah, V., Gross, R. A. Supramolecular Assembles of a Naturally Derived Sophorolipid. Langmuir; 20; 7926-7932 (2004).
-
Singh, S,K, Felse, A. P., Nunez, A., Foglia, T.A. and Gross, R.A. Regioselective Enzyme-Catalyzed Synthesis of Sophorolipid Esters, Amides and Multifunctional Monomers. J. Org. Chem.; 68; 5466-5477 (2003).
-
V Guilmanov, A Ballistreri, G Impallomeni, R.A. Gross, “Oxygen Transfer Rate and Sophorose Lipid Production by Candida bombicola”, Biotechnol. and Bioeng; 77(5), 489-494 (2002).
-
K.S. Bisht, W Gao, R.A. Gross, “Glycolipids from Candida bombicola: Polymerization of 6-O-Acryl Sophorolipid Derivative”, Macromolecules, 33, 6208-6210 (2000).
-
K. Bisht, R. Gross and D. Kaplan, “Enzyme-Mediated Regioselective Acylations of Sophorolipids”, J. Org. Chem., 64:3, 780-789 (1999).