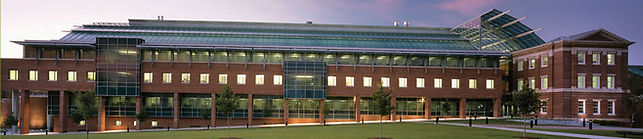


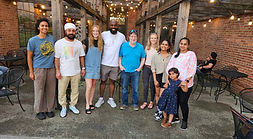

Methods for conversion of fatty acids to w-hydroxy acids, ω-carboxyacids, oligomers and polymers.
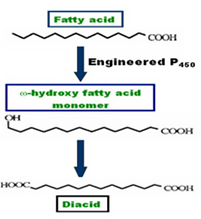
An engineered Candida tropicalis strain was developed to do specific oxidation at the w-position of agriculturally derived fatty acids. This new synthetic route to transform fatty acids to their corresponding ω-carboxyl and w-hydroxyl fatty acids allows the facile synthesis of difunctional fatty acids for use as polymer building blocks and other specialty applications. Critical to success in this research has been work performed in collaboration with DNA 2.0 to engineer C. bombicola so that pathways involved in up-oxidation of w-hydroxyl fatty acids are blocked. Furthermore, P450 engineering is enabling conversions of fatty acid substrates that previously were poor substrates. A wide range of α,ω-dicarboxylic acids with different carbon lengths (C12-C22) and internal functionality (e.g. alkene, alkyne, hydroxyl and epoxy) were prepared. The fermentative conversion of oleic acid to cis-9-1,18-octadecenedioic acid was scaled-up giving high volumetric yields. Furthermore, a method for downsteam processing was developed that enables the facile recovery of product in high purity. Lipase-catalyzed condensation polymerizations were used to create a versatile family of functional polyesters from these ‘fatty diacid’ building blocks. For the first time, high levels of ω-hydroxyfatty acids were prepared via microbial fermentation.
Scheme 2. Engineered Candida
strains as catalyst to transform
fatty acids to their corresponding
ω-hydroxy and ω-carboxy fatty acids

Scheme 3. Lipase-catalyzed polycondensation reactions between non-activated diacids and diol monomers that vary in chain length.
Establishing methods for enzyme-catalyzed condensation polymerizations
A fundamentally important class of polymerizations in polymer chemistry are polycondensations between monomers bearing hydroxyl and carboxyl functionalities. Tradition routes to such condensation polymerizations involve organometallic catalysts and high-temperatures (≥ 150 oC). The use of enzymes in place of traditional catalysts can lower reaction temperatures, reduce side-reactions, enable the use of monomers that are thermally sensitive, and provides opportunities to exploit enzyme stereo- and regioselectivity. Towards this end, our laboratory has made significant contributions towards development of lipase-catalyzed-step-condensation polymerizations between non-activated diacids and diols. The majority of previous work focused on activated acid monomers that are impractical due to cost considerations.
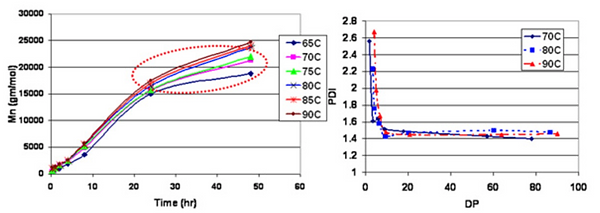
Figure 1. Effect of reaction temperature: in-bulk I-CALB catalyzed adipic acid/octane diol polymerization.
Of interest upon initiating this work was whether lipase-catalysts would exhibit control during chain growth in product characteristics such as end-group and polydispersity. In one study (Mahapatro et al., 2004a), we focused on condensation polymerizations between adipic acid and 1,8-octanediol. Polymerizations were conducted in the absence of solvent and without use of groups that activate monomer carboxylic acids. Under a wide range of reaction conditions and without fractionation, products formed had Mw/Mn values ≤ 1.5 (Fig 1). Such low polydispersity values demonstrate that CALB catalyzes chain growth with chain-length selectivity. This ability of CALB to form products of substantially lower polydispersity than would have been attained by conventional chemical catalysts is an important attribute of CALB-catalyzed condensation reactions. Growth of polymer chains was found to be nearly invariable for reaction temperatures from 65 to 90 °C (Fig 1). Two contributing factors are diffusion constraints that limit further chain growth and that CALB reduces the activation energy of the rate-determining step so that increases in temperature have less effect on rate. Another study reported the successful use of CALB catalysis for polymerizations of linear aliphatic hydroxyacids (Mahapatro et al., 2004b). Polymerizations were performed in bulk, at 90 °C, using 10% w/w relative to monomer of Novozym 435 (macroporous beads that contain 10% w/w of lipase B from Candida antarctica). There was little difference in the time profile of DPavg values for w-hydroxyacids with chain lengths of C-16, C-12, and C-10. These polymerizations gave products with DPavg about 105 and Mw/Mn of 1.5 by 24 h. However, shorter chain substrates (e.g. 6-hydroxyhexanoic acid) polymerized relatively slower. The apparent insensitivity to w-hydroxyacid chain lengths between C-10 and C-16 was surprising given the general chain-length selectivity of lipases. Similar to Novozyme-435-catalyzed diacid/diol polycondensations, Mw/Mn values tended toward 1.5 as polymerizations progressed. This result is in contrast to Mw/Mn values of about 2.0 for Novozyme-435-catalyzed lactone polymerizations. Fundamental aspects of the polymerization mechanism that lead to these very different polydispersity values are not yet understood. Plausible explanations are that polydispersity values of lactone polymerizations are broadened by slow chain initiation and a greater tendency (than in condensation polymerizations) to undergo transesterification reactions. Nevertheless, the narrow dispersity of polymers from Novozyme-435 condensation polymerizations can provide beneficial solid-state properties such as enhanced crystallization rates.
References
-
Sahoo, B; Bhattacharya, A.; Fu, H.Y.; Gao, W.; Gross, R.A. Influence of PEG end-group and molecular weight on its reactivity for lipase-catalyzed polyester synthesis BioMacromolecules 7 (4): 1042-1048 (2006).
-
Azim, H.; Dekhterman, A.; Jiang, Z.Z.; Gross, R.A. Candida antarctica lipase B-catalyzed synthesis of poly(butylene succinate): Shorter chain building blocks also work, BioMacromolecules 7 (11): 3093-3097 (2006).
-
Mahapatro, A.; Kumar, A.; Kalra, B.; Gross, R. A. “Solvent-Free Adipic Acid/1,8-Octanediol Condensation Polymerizations Catalyzed by Candida antarctica Lipase B” Macromolecules; 37(1); 35-40 (2004a).
-
Mahapatro, A.; Kumar, A.; Gross, R. A.; “Mild, Solvent-Free-Hydroxy Acid Polycondensations Catalyzed by Candida antarctica Lipase B.” BioMacromolecules; 5(1); 62-68 (2004b).
-
Mahapatro, A.; Kalra, B.; Kumar, A.; Gross, R. A.; Lipase-Catalyzed Polycondensations: Effect of Substrates and Solvent on Chain Formation, Dispersity, and End-Group Structure BioMacromolecules; 4(3); 544-551 (2003).